Vertebrates are modified segmented worms; that is, their body plan is made up of sequentially repeated units, most apparent in skeletal structures like the vertebrae.
Arthropods are also modified segmented worms. Look at a larval fly, for instance, and you can see they are made up of rings stacked together.
So here’s a simple and obvious question: can we infer that the last common ancestor of vertebrates and arthropods was also a segmented worm? That is, is segmentation a common ancestral trait, or did arthropods and vertebrates invent it independently? At first thought, you might assume they are: it’s a complex trait shared by two taxa, so the simplest assumption is that both groups inherited it from their common ancestor (making it a synapomorphy), but there are also substantial differences in the mechanism of segmentation, so it’s possible that this trait wasn’t present in the common ancestor (making it a homoplasy).
Which is it? And the answer is…we don’t know! There’s a great deal of sympathy for synapomorphy, driven largely by a molecular bias — we see that a lot of the genes involved in the process in both vertebrates and arthropods are shared. There is a whole family of Notch-related cyclic genes, for instance, that turn out to be important in both, but the catch is that Notch is a gene that gets recruited in all kinds of processes — it’s part of a handy developmental module for defining borders. So its presence doesn’t automatically imply homology.
And then there’s the whole problem of segmentation looking so different in flies (weird, highly derived arthropods) and vertebrates.
Flies build their segments almost all at once. In the fly embryo, there is first a broad gradient of position information, then a set of genes called the gap genes are switched on to define broad zones in the animal, and then, finally, the segmentation genes read the pattern of the gap genes and interact with each other to partition the fly into segments. It means segmentation is fast: all 14 segments form in one short interval, nearly simultaneously.
There’s another complication. The segmentation genes in flies are numerous and elaborate, and in particular, they set up a peculiar pattern of alternating periodicity using genes called pair-rule genes. That means that there is one set of genes active in all of the odd-numbered segments (eve, or even-skipped, for instance), and a different set active in all of the even-numbered segments (ftz, or fushitarazu). In addition, there’s a regular platoon of other pair-rule genes like odd and prd and hairy that are expressed in alternating segmental domains.
What it means is that fly segments alternate in their molecular substrates, like the floral wallpaper to the right.* It seems excessively complicated. Furthermore, when we look at the molecular circuitry of segmentation, it’s surprising inelegant — each stripe is hard-coded into the regulatory controls of the genes involved. The ugliness of evolutionary contingency is on full display here.
When we look at vertebrates, on the other hand, we see something very different. Segments form sequentially, one at a time from front to back, rather than nearly simultaneously. What we see here is a clock-and-wavefront model in operation, where cyclic surges in the expression of two molecules, fgf8 and Wnt3a, trigger a small pool of cells at the front of an undifferentiated band to pinch off and differentiate into a segment. Then the wave recedes, the front of the undifferentiated band matures a little more, and then when the wave rises again, another set of cells are recruited to make the next segment. And so on and on, until the whole band is delineated into an array of segments.
We find many of the same genes seen in flies involved in this process; for instance, a homolog of the Drosophila pair-rule gene hairy is found in vertebrate segmentation. However, vertebrates don’t have the alternating pattern of segmentation — it’s the same genes used in each.
Now you’re thinking that maybe segmentation evolved independently in vertebrates and arthropods, because I’ve just highlighted all these major differences. But wait! There’s a complication!
Most of what we know about arthropod segmentation comes from Drosophila, and I’ll say it again: flies are weird, highly derived, extensively modified to a point where it’s surprising they have anything in common with their pre-Cambrian ancestors anymore. All that stuff about near-simultaneous formation of the segments seems to be a product of selection for fast development in flies — those are all recent additions to their developmental programs. We may be getting misled by modern additions to improve the efficiency of the fly-making factory, and so we aren’t seeing the original arthropod segmentation plan at all.
To do that, we need to look at an insect that doesn’t use this highly derived Drosophila mechanism, and that’s easy: there are a lot of insects other than flies out there. Tribolium castaneum, the flour beetle, is a popular alternative. These animals do not form their segments all at once, but instead have a growth zone from which segments differentiate sequentially, from front to back…hey! Like a vertebrate! At least, like a vertebrate superficially — do they also use a clock-and-wavefront mechanism, with periodic surges of signaling gene expression, as vertebrates do?
The tentative answer to that question is yes. Recent observations show that some genes do show clock-like changes in the level of expression in the growth zone.
In the Tribolium embryos below, all were stained for RNA of the pair-rule gene Tc-odd, and you can clearly see the nice segmental stripes of blue Tc-odd RNA in the segmented bits. The interesting part is the posterior growth zone (gz), marked with the gray box in the plots of stain intensity. What you should see in these specimens fixed at different times is a changing level of Tc-odd expression in the gz — do enough of these, and you get a good impression of rising and falling levels of Tc-odd expression over time.
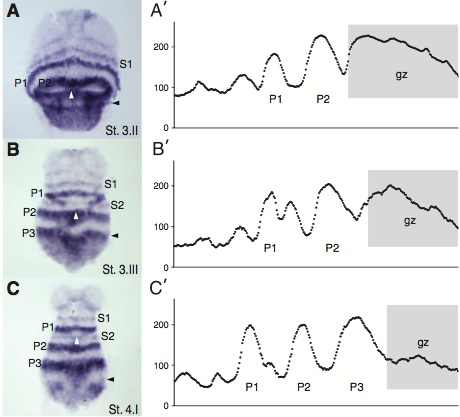
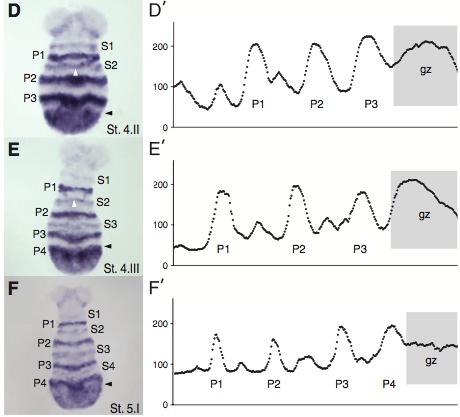
Expression of Tc-odd during early germband elongation. (A to F) In situ hybridization for Tc-odd in elongating germbands at stages 3.II to 5.I, in ventral view (for staging, see supporting online material). The growth zone is located posterior to the black arrowheads. White arrowheads mark the posterior edge of the amniotic fold. (A′ to F′) Intensity profiles of Tc-odd expression along the anterior-posterior axis of germbands. The intensity of staining was measured on the lateral part of the germbands shown in (A) to (F), encompassing the ectoderm and the amnion (for explanations, see fig. S1). Note the changing profile of Tc-odd expression within the posterior growth zone (gray area) during the emergence of new primary Tc-odd stripes from that zone. During phase I of stripe formation [(C) and (F)], the growth zone has low levels of Tc-odd, except for two lateral spots of expression that mark the initiation of a new cycle of Tc-odd expression. At phase II [(A) and (D)], Tc-odd expression extends through the growth zone, such that a posterior domain of high Tc-odd expression is established. At phase III [(B) and (E)], Tc-odd expression in the posterior-most part of the growth zone is reduced to low levels, whereas cells at the anterior of the growth zone still express high levels, establishing a broad primary stripe. In a typical collection of embryos, each phase is found in roughly equal numbers. P1 to P4, primary stripes; S1 to S4, intercalating secondary stripes; gz, growth zone.
That isn’t a perfect experiment on its own, though. There’s variability in the degree of expression of Tc-odd from animal to animal and experiment to experiment, and timing the embryos is tricky and with the possibility of error; what you really want to do is see the changing expression in living embryos. They don’t have that assay in Tribolium, yet, but the investigators did something clever instead. They cut embryos in half.
The bifurcated embryos continue developing normally for some hours afterwards, so what you can do is cut them in half, fix one half immediately, let the second half continue developing for some time afterwards, fix it, and then stain both for Tc-odd. Now you can make a direct comparison of the change in gene expression in the same embryo at two different times in development.
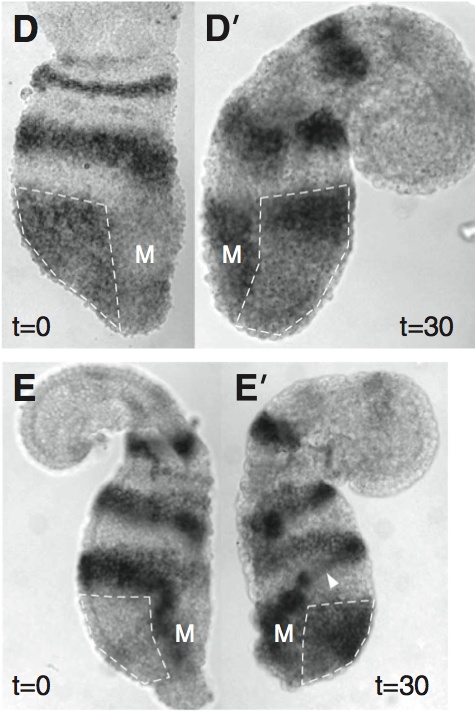
(D and D′) In situ hybridization for Tc-odd on two halves of the same embryo. One half was fixed immediately after dissection (D), whereas the other was cultured for 30 min at 30°C before fixation (D′). Tc-odd expression in each half-embryo is at phase 3.II and 3.III, respectively. (E and E′) Same experiment, with half-embryos at phases 4.I and 4. II. The lateral part of the growth zone, encompassing the ectoderm and amnion, is outlined with a dashed line; the medial part, which also includes mesoderm, is marked M (see fig. S1). In panel E′, the oblique stripe of Tc-odd expression (white arrowhead) is in the amnion. Anterior is toward the top in all panels.
It’s clear. There is a kind of segmentation clock running in Tribolium, and so now you might be leaning back towards thinking it’s reasonable to assume that this mechanism might have been inherited from the last common ancestor of both vertebrates and arthropods.
But hang on, we still have unresolved issues. Tribolium also exhibits the alternating segment periodicity we see in Drosophila, but don’t see in vertebrates. In fact, when you look at the whole network of segmentation genes in Tribolium it does still look a lot like the fly segmentation network…but not so much like the vertebrate network.
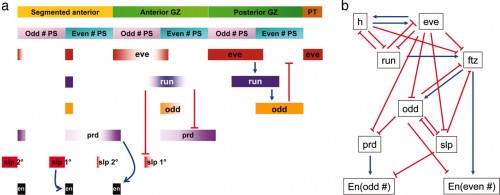
Pair-rule patterning in Tribolium. (a) The dynamic expression of the primary and secondary pair-rule genes and their regulatory interactions are summarized. The bar at the top indicates that anterior is to the left. Newer segments forming in the growth zone are to the right. In this model of pair-rule patterning in Tribolium, two-segment units are prepatterned in the posterior region of the growth zone through one cycle of the regulatory circuit (Tc-eve, Tc-run, and Tc-odd). As the expression of Tc-run retracts anteriorly in even-numbered parasegments, the expression of Tc-prd is derepressed. Primary Tc-prd stripes resolve into two secondary stripes, showing alternatively weak and strong segmental expression. The strong secondary stripes in odd-numbered parasegments regulate Tc-En expression. Tc-run also retracts posteriorly in odd-numbered parasegments, resulting in derepression of the primary Tc-slp stripes. As Tc-run expression fades, expression of the primary Tc-slp stripe extends to the posterior border of the odd-numbered parasegment, which is required for the activation of Tc-En. GZ, growth zone; PS, parasegment; PT, posterior tip. (b) The more complex pair-rule network in Drosophila.
Aaargh, so maybe segmentation evolved independently in insects and vertebrates after all?
But wait again…there are big differences between Tribolium and Drosophila. Genes interact differently, and different genes have subtly different roles in setting up the pattern.
In Drosophila, the three primary pair-rule genes (h, eve, and run) are key players in initiating pair-rule patterning. However, Tc-h seems not to function as a pair-rule gene at all. Although odd is a secondary pair-rule gene in Drosophila that is repressed by eve, Tc–odd functions as a primary pair-rule gene in Tribolium that represses Tc–eve. Repression of slp and odd by eve is critical to activate prd-dependent odd-numbered and ftz-dependent even-numbered en stripes, respectively, in Drosophila In contrast, Tc–eve is required for the activation of Tc–odd, which in turn represses Tc–eve to prepattern a two-segment unit. Furthermore, Tc–run, which is induced by Tc–eve, is important for the formation of Tc–prd-dependent odd-numbered and Tc–slp-dependent even-numbered Tc–en stripes. Drosophila ftz is a secondary pair-rule gene that activates even-numbered en stripes, but Tc–ftz does not function in segmentation. Differences in the primary pair-rule genes result in different genetic interactions between primary and secondary genesand likely affect the regulatory interactions between pair-rule and segment polarity genes. For example, loss of slp affects odd- numbered parasegments, whereas loss of Tc–slp affects even-numbered parasegments.
What this tells me is that this tangled web of interacting gene products is robust and flexible; it can be tweaked by evolutionary changes and still function to produce a reliable segmental pattern. It’s also the kind of module that has a core circuit, and has accumulated lots of supplemental inputs that can reinforce the segmental pattern generation, and can also be swapped in for core elements — flies use h–eve–run as the primary patterning genes, while beetles use eve–run–odd, so the actors can shift about in their roles while still producing a recognizably similar play.
Also, these similar networks can function in very different settings: I’m surprised to see the same core players in the all-at-once segmentation pattern in flies and the one-at-a-time sequential segmentation of beetles. What we need is much more comparative molecular data on segmentation networks so we can identify the minimal segmentation core and how it works. Then we have a chance of determining when that basic module evolved, and where it belongs in phylogeny.
Until then, we don’t know whether segmentation is a synapomorphy or a homoplasy between vertebrates and arthropods, which is actually kind of cool. Mysteries in science are waiting to be resolved, and the data is on the way!
Choe CP, Miller SC, Brown SJ (2006) A pair-rule gene circuit defines segments sequentially in the short-germ insect Tribolium castaneum. Proc Nat Acad Sci USA 103(17):6560-6564.
Sarrazin AF, Peel AD, Averof M (2012) A segmentation clock with two-segment periodicity in insects. Science 336:338-341.
*Yeah, that’s the hideous wallpaper in my bedroom, put there in the 1950s, I think. My only excuse is that almost all the time I spend in that room either it’s dark or my eyes are closed.
Thanks PZ. This post is one of the reasons I keep coming back here.
I am trained as an immunologist, but I loves me some developmental biology for breakfast.
NOM NOM NOM.
I was taught that the chordates are most closely related to the echinoderms, which obviously have nothing like the annelid/arthropod body plan. Does that not argue that arthropod segmentation is evolutionarily independent of vertebrate segmentation?
Common design makes it all so much easier, not to mention so much more stupid.
Glen Davidson
I kings 3 verse 24 to 28
A sword was brought, and Solomon ordered, 25 “Cut the Embryo in half! That way each half will be used to show developmental biology in Arthropods.
26 “Please don’t kill my son,” the baby’s mother screamed. “Your Majesty, I love him very much, but give him to her. Just don’t kill him.” The other woman shouted, “Go ahead and cut him in half. Then neither of us will have the Tribolium embryo.”
27 Solomon said, so what you can do is cut them in half, fix one half immediately, let the second half continue developing for some time afterwards, fix it, and then stain both for Tc-odd. Now you can make a direct comparison of the change in gene expression in the same embryo at two different times in development.
28 Everyone in Israel was amazed when they heard how Solomon had made his decision. They realized that God had given him wisdom to judge fairly.
This is ignoring that all the basal, non-chordate deuterostomes aren’t segmented. Also, the phylogeny of Ecdysozoa isn’t well worked out, but if priapulids and nematodes branch off separately first, that also argues against their common ancestor being segmented.
So where does annelid segmentation fit into all this?
IIRC, echinoderms actually are segmented, kinda. Each arm is a different embryonic segment, and the adult form is a curling of the segmented worm into a circle….
What’s with all the science?! Where’s the leftleaning atheïsm?!
/snark
I have so many tabs open now that I need to (re)read before trying to comprehend all this again.
I’m not a biologist by any means – quite a bit of that was over my head – but I’ve often wondered about homology between vertebrates and invertebrates.
Oddly, enough, this curiosity came from watching the remake of The Fly – I remember thinking that there’s no way that Jeff Goldblum’s arms and legs are homologous to the front and back legs of an insect. Not that that was the most glaring liberty taken with biology in that movie…
If I’m not mistaken*, the Hox** family complex evolved in the ancestor of bilateria. Given that these genes are expressed colinearly in all bilaterians, doesn’t this argue that the bilaterian ancestor was at least differentiated along the posterior/anterior body axis?
So if not a segmental worm, something with serial differentiation and bilateral symmetry, right? Are these distinct?
My big question is, in the ancestor of the bilaterians, did the blastopore develop into the mouth or the asshole?
**I probably am.
*Orthologues exist in other taxa, IIRC, but aren’t, you know…complexed.
If we’re descended from worms, why are there still worms?
Let me join the chorus that bemoans the lack of a phylogenetic perspective. Keep in mind that the last common ancestor of flies and vertebrates was also the last common ancestor of flatworms and Xenoturbella.
Further, keep in mind that different organ systems are segmented in vertebrates and arthropods (and different ones again in annelids). In vertebrates, the body-wall musculature is segmented; in arthropods it’s not. In vertebrates, there’s a part of the body that lies in front of the first segment (basically the eyes and everything in front of them); in arthropods, the body apparently starts with the first segment, which carries the eyes and originally the mouth (which has now bent downwards, so in insects it opens behind the third segment). In vertebrates, the pharynx is segmented – but these segments don’t even try to match up with those of the body-wall musculature! (No comparison to arthropods can be made; arthropods lack gill slits.) In vertebrates, the skin is not segmented – scales (including gastralia) practically never line up with ribs; in arthropods it (or anyway the cuticle which vertebrates lack) is segmented. In vertebrates, the kidney originally participates in the segmentation of the body-wall musculature; in arthropods, that’s AFAIK not the case.
Ouch, it causes whip lash back to a prior life when I did these kinds of experiments (though with the tech of a decade ago).
Did you see this paper from Steve Small’s lab that just came out in Cell?
http://www.ncbi.nlm.nih.gov/pubmed/22541432
It shows that bicoid gradient-dependent target-gene boundary formation is dependent on an opposing gradient of repressors, including runt pair-rule gene, which is read out by the bicoid-dependent enhancers in the target genes. I am not an expert on this shitte, but my understanding is the existing theory was that the bicoid-dependent enhancers were threshold elements that directly read out the bicoid gradient. This obviously requires revision of that theory.
Thanks, PZ! Please keep the evo devo posts coming.
Heh, sorry, I was thinking of “fish” scales, bone plates in the dermis that have been independently lost by amniotes, lissamphibians and not many other clades. In snakes, the purely epidermal scales line up with the ribs and vertebrae, because all of these together are used for locomotion; the epidermal scales of other squamates, tuatara or crocodilians don’t line up with the skeleton.
Your question is wrong. Flatworms still lack separation of mouth and asshole, and so, IIRC, does Xenoturbella (I’m too lazy to look this up at half past 2 in the morning).
Incidentally, both arthropods and vertebrates have both a stomodaeum and a proctodaeum; the blastopore closes soon after gastrulation. But that’s clearly not homologous. :-)
I totally love this. Even if I’m pretty sure understand only a tiny segment of the post.
A scigasm? Was it good for you?
First mrbongo rants about “moderate” Islam, and THEN it mentions Pakistan.
That alone, tells us it is an intellectually dishonest, pitiful, unethical excuse for a human being, with no interest in actual honest discussion.
At least if it had said Singapore, and country where Islam actually is moderate, it would have had just a teensy bit more credibility.
But PZ, think about the Tone! you’re putting out all this hard science, with (gasp!) facts, and detailed information! What next, physics ? Seriously though, great post. one of the things that drew me to Pharyngula back in the day it was on sciblogs.
@Physioprof (14) what, you mean science actually correcting itself? W T F ? you mean it’s not instantly carved in stone?
How can there be proper dogma when it’s not Carved in Stone ?
Dammit man, how can we ever get Scientism off the ground as a religion with no dogma ?
The shit they must say ;)
90% of vertebrate tax class (as I recall) was learning the location of the anus. The othe 10% was gonads, but that was pretty easy…in most inverts, gonads are everywhere.
Is there a poll? Which side of the question are the hordes piling on?
Is anyone doing this kind of work with myriapods and basal hexapods (diplurans and such)? – it seems bleeding obvious that flies and beetles are not going to tell us much about ancestral states.
(I was going to say something about dermal-vertebral correlation in snakes, but too late, never mind…)
Yep, Xenoturbella has “no through gut”.
And if you need your mind blown for a minute, take a look here. I don’t believe it, but I’d need to study the methods in depth and lack the time.
ChasCPeterson did in the Sb thread that PZ forgot to link to. :-)
Actually, no. The miRNA dataset actually supports Xenoturbella not being a bilaterian but the sister-group of Acoelomorpha, and the sequence-based datasets lead to scarily short internodes that should be red flag. I think peer review was a bit light on this paper.
Or onychophorans, tardigrades, basal crustaceans (whichever those are)…
The dermal-vertebral correlation in snakes seems to me to be pretty obviously a derived trait that is the way it is because of functional reasons and selective pressures pertaining to that, and cannot be reliably considered to have any bearing on ancestral anatomy.
OK, I really want to learn more so I can understand what I’m reading here.
Would one (or more) of you be so kind as to suggest how to proceed? I’ve taken college-level courses in human anatomy and physiology, and I think I have a decent understanding of vertebrate anatomy in general.
What book or courses would be most help to the educated layperson?
Thanks.
There are a good number of specifying genes/molecular correlates to head segments seen in D. melanogaster. Though they are certainly understudied, and some additional relevant genes on one of the major chromosomes probably remain unknown because their mutants were sorted out of the major systematic screen.
The role of bicoid in anterior gradient formation in the D. melanogaster syncytial blastoderm is famous, but less known is that the cloning paper showed presence of a smaller, later expressed mRNA isoform that afaict has never been studied. The mammalian homologs to bicoid are PITX1/2 with expression in the mnteriormost embryonic ‘ectomere’ and mutant defects primarily in upper lip and nasopharyngeal tissues. Which together suggests that the ancestral bicoid/Pitx family gene function serves to specify the first, putative clypolabral segment/field.
A conference I went to in the Nineties had a French scientist present who misexpressed orthodenticle protein in the head region of an empty spiracles mutant in early Dm embryos and Ems protein in the head region of Otd mutant embryos. He got convincing replacement of the deuterocerebrum by a second protocerebrum in the first, protocerebrum by a second deuterocerebrum in the second. Regrettably I don’t think he ever published, for some reason. The developmental stall, head structure muddling, and lethality was such that no one asked whether ectopic eyes or antennae were coinduced. But that seems like evidence for homeotic transformations of two more segments or fields and identification of homeobox genes that are their primary specifiers. The tritocerebrum specifying gene product, which possibly also specifies the intercalary segment and headcase in insects, is apparently still an area of active investigation.
Otd and Ems were picked up in the Nüsslein-Volhard/Wieschaus screen not so much because of their fairly subtle, though lethal, head defects but because of much more visible phenotypes of tracheal defects in the abdomina and denticles. Mutants in a third homeobox gene, Vvl, likewise links (rather minor) head defects with tracheal defects. The LCA perhaps linked induction of some head structures with associated gill openings or gill/tracheal structures and tissues.